

For instance, while both group Ia and II afferents relay changes in static muscle length, the dynamic sensitivity of group Ia afferents enables them to also signal the rate of change in muscle length 14, 15. Despite supplying the same sensory end organ, group Ia and group II afferents exhibit distinct intra-spindle innervation patterns, activation thresholds, and conduction velocities, features that appear to render them biased to qualitatively different information of muscle stretch. Unique among sensory organs, MSs are subject to (CNS-directed) efferent motor control through dynamic and static gamma motor neurons (γMN) which innervate the contractile polar ends of the intrafusal muscle fibers and effectively set the gain for group Ia/II afferent discharge frequency 12, 13. muscle length) result in increased or decreased firing rates 11. Both types of afferents are responsive to stretch of the intrafusal fibers, such that voluntary or passive changes in limb position (i.e. They are embedded within the belly of skeletal muscles and consist of encapsulated intrafusal muscle fibers that are typically innervated by one primary (group Ia) and several secondary (group II) proprioceptive sensory neurons (pSNs) (Fig.
MUSCLE SPINDLE AND GOLGI TENDON ORGAN DRIVERS
Muscle spindle (MS) mechanoreceptors are considered the main drivers for the sense of limb position and movement (kinesthetic sense) 8, 9. Extensive anatomical and physiological analysis have revealed two types of muscle receptors: muscle spindles and Golgi tendon organs 7. Proprioceptive feedback derives in large part from specialized mechanoreceptive organs in skeletal muscle. At present, such studies remain challenging due to the lack of genetic access to individual proprioceptor subtypes. Many decades of study of the intramuscular sense organs and their afferent innervation have led to predictions on how proprioceptive feedback integrates with other sensory modalities to influence central motor circuits and calibrate motor output, yet most of these inferences await further corroboration in behaving animals 4, 5, 6. Collectively referred to as the proprioceptive sense, this afferent information informs the central nervous system (CNS) on the position of the body and limbs in space 1, 2, 3. Sensory feedback from muscle, skin, and joints is critical for the normal execution of voluntary motor tasks. Together, our data provide comprehensive molecular signatures for groups Ia and II MS afferents and group Ib GTO afferents, enabling genetic interrogation of the role of individual proprioceptor subtypes in regulating motor output. Lineage analysis between proprioceptor transcriptomes at different developmental stages provides evidence that proprioceptor subtype identities emerge late in development. Validation of cluster-specific transcripts in dorsal root ganglia and skeletal muscle demonstrates that two of these clusters correspond to group Ia MS afferents and group Ib GTO afferent proprioceptors, respectively, and suggest that the remaining clusters could represent group II MS afferents.

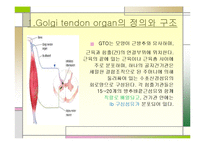
We performed single cell RNA sequencing of genetically identified adult proprioceptors and uncovered five molecularly distinct neuronal clusters. Proprioceptive feedback mainly derives from groups Ia and II muscle spindle (MS) afferents and group Ib Golgi tendon organ (GTO) afferents, but the molecular correlates of these three afferent subtypes remain unknown.
